
Research
Our group studies the structure and function of macromolecular assemblies using electron cryomicroscopy (cryo-EM), image analysis, molecular biology, and molecular genetics. We also develop the tools of cryo-EM so that we can answer questions that are not amenable to the techniques that currently exist. This process occurs at the level of developing new algorithms and software for image analysis, performing calculations with images and models of molecular structure, and developing new methods for specimen preparation.
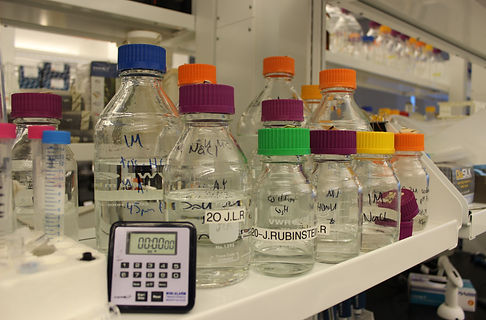
RESEARCH DIRECTIONS
Cryo-EM method development and Bioenergetics
Electron cryomicroscopy (cryo-EM) of macromolecular assemblies has become an important technique in structural biology. The method allows biologists to determine high-resolution structures of proteins that cannot be crystallized for X-ray crystallography, and also allows for investigation of the molecular motions that are essential for biomolecular function. We are developing new methods for preparing specimens, imaging them, and performing image analysis in order to expand the potential of the technique.
​
We are particularly interested in the control and flow of energy in biological systems (bioenergetics). In biology, chemical energy is frequently interconverted with transmembrane electrochemical gradients of protons. In humans and some pathogenic bacteria, the supply of energy from metabolism is dependent on the availability of the electron acceptor oxygen. Our structural studies aim to understand, at a molecular level, processes such as
-
Mycobacteria oxidative phosphorylation as a target space for tuberculosis (TB) and nontuberculosis mycobacterial (NTM) drug discovery
-
Control of pH in intracellular compartments by the V-type ATPase
-
Loading of neurotransmitters into synaptic vesicle by V-type ATPases
ATP synthase
The ATP synthase is the central enzyme in biological energy metabolism. Following the Nobel prize winning determination of the structure of the catalytic F1 region by the group of John Walker in 1994 using X-ray crystallography, we determined the first high-resolution structures of the ATP synthase membrane region in 2017 using cryo-EM. We also resolved the rotational states of the enzyme (2015), showed how the structure deforms under strain (2022), and determined the first high-resolution structure of a bacterial ATP synthase (2019). Our work on ATP synthase now relates to the mycobacterial enzyme, which is the target of the important TB drug bedaquiline and several other experimental compounds.
Electron transport chain
The electron transport chain complexes establish the transmembrane proton motive force that powers ATP synthesis in mitochondria and aerobic bacteria such as the pathogenic mycobacteria that cause tuberculosis. Despite decades of study, it is unclear how the passage of electrons through the electron transport chain complexes, which ultimately leads to the reduction of oxygen to water, powers proton transport. Much of our work on the electron transport chain relates to understand the process in mycobacteria to understand how it interacts with different metabolic pathways and allows pathogens to survive in a variety of environments.
V-ATPase
Vacuolar-type ATPases are ATP-powered proton pumps that control the pH of numerous intracellular compartments in all eukaryotic cells and the extracellular environment of some specialized cells. These enzymes have important roles in endocytosis, exocytosis, bone maintenance, kidney function, neurological disorders, and cancer. We study the enzyme from yeast and mammalian cells to understand the fundamental properties of its assembly and function, and to understand how it helps control the loading of synaptic vesicles with neurotransmitters in neurons.
Other
In addition to the projects listed above, there are a number of exciting new bioenergetics and collaborative directions being explored in the laboratory.
RESEARCH INFRASTRUCTURE
The laboratory is housed on the 20th floor of the state-of-the art Peter Gilgan Centre for Research and Learning at the Hospital for Sick Children and is equipped with extensive infrastructure for cryo-EM. We are also involved in developing new hardware and testing new instruments developed by established manufacturers and startup companies. John Rubinstein is the director of the Hospital for Sick Children's Nanoscale Biomedical Imaging Facility. Major infrastructure available for research include:
-
A ThermoFisher G3 Titan Krios with Falcon4i direct detector device camera and SelectrisX energy filter. Facility staff are closely integrated in our research group and we help ensure this instrument performs at highest possible level for the entire Toronto biomedical research community
-
A ThermoFisher Scientific Glacios 2 with Falcon 4i camera. This instrument serves The Hospital for Sick Children and Lunenfeld-Tanenbaum Research Institute
-
Two Hitachi HT7800 120 kV room temperature TEMs for negative stain EM
-
ThermoFisher Scientific Vitrobot grid freezing devices
-
Leica EM GP2 grid freezing device
-
Gatan CP3 grid freezing device
-
PELCO EasiGlow glow discharge devices
-
A variety of sputter coaters and carbon evaporators
-
Raise3D Pro 3D printer
-
Extensive computational infrastructure (multiple servers, RAIDs, and GPU nodes)